Dieser Beitrag ist auch verfügbar auf:
Deutsch
The ATP and the microcurrent – adenosine triphosphate
Summary for readers in a hurry.
The molecule ATP is an important energy store and distributor in the body. It is used in various cellular processes such as muscle contraction, protein biosynthesis, nerve transmission and active transport processes. ATP releases energy by cleaving the phosphate bond. It is one of the most energetic phosphates in the body.
Microcurrent therapy increases the production of ATP in the tissues and thus accelerates the healing process. ATP is crucial for wound healing as it is needed for the synthesis of proteins, phospholipids and other chemical compounds. It also plays an important role in nerve transmission and active transport across cell membranes.
Microcurrent therapy uses a low-intensity pulsed direct current that increases ATP production. Unlike other forms of electrotherapy, microcurrent therapy has a cumulative effect and can charge the tissue with ATP. This accelerates tissue healing, reduces inflammation and swelling and increases physical resilience.
Microcurrent therapy is more effective than other forms of electrotherapy with higher amperages because it increases the amount of ATP in the tissues. ATP production is stimulated by the flow of current between the electrodes, creating an environment of negative hydroxyl ions at the cathode and protons at the anode. The protons diffuse through the tissue and lead to increased ATP formation.
ATP is the body’s energy currency and plays a crucial role in various cellular processes. Microcurrent therapy increases ATP production and thus accelerates the healing process. The application of microcurrent can increase the concentration of ATP in the tissues, leading to improved tissue healing and other positive effects.
Detailed explanation of ATP production and microcurrent therapy
.
ATP (adenosine triphosphate) molecules are the storage and distribution vehicles for energy in the body. Energy is obtained when ATP is split into ADP. It is the cleavage of the phosphate bond that provides the energy. ATP is one of the most energy-rich phosphates in our body.
This energy is used in almost all energy-related cell reactions. Not only is ATP an essential part of the function of virtually every cell in our body, but we can also look at ATP function by category of activity. These essential functions include:
- Muscle contraction;
- Protein biosynthesis;
- Nerve transmission; and
- Active transport across cell membranes.
In muscle contraction, the process is as follows: Each muscle spindle is made up of muscle fibres. Inside the muscle fibres are many muscle fibrils. These muscle fibrils are in a fluid matrix, the sarcoplasm. In the sarcoplasm are thousands upon thousands of mitochondria that contain large amounts of ATP.
It is ATP that drives the muscle contraction process through the ATPase activity of the exposed myosin head. When exposed to the myosin head, ATP is cleaved and energy is released. It should be noted that in addition to ATP, magnesium is also very important for ATP energy release reactions. Before ATP can become “active ATP”, magnesium must be bound between the second and third phosphate. Clinically, magnesium deficiency can be associated with conditions such as fibromyalgia and chronic fatigue syndrome.
The synthesis of almost any chemical compound requires energy. This energy is ATP, which is crucial for the biosynthesis of proteins, phospholipids, purines, pyrimidines and hundreds (if not thousands) of other substances. Take the involvement of ATP in protein synthesis as an example: a single protein can consist of many thousands of amino acids. In order to link two amino acids together, four energy-rich phosphate bonds have to be cleaved.
A maximum of two ATP could serve as energy for the connection of two amino acids. So if our protein is made up of 10,000 amino acids, it may take 20,000 ATP to form just that one protein. It should also be noted that the amino acids themselves indirectly consume ATP as they are first transported into the cells.
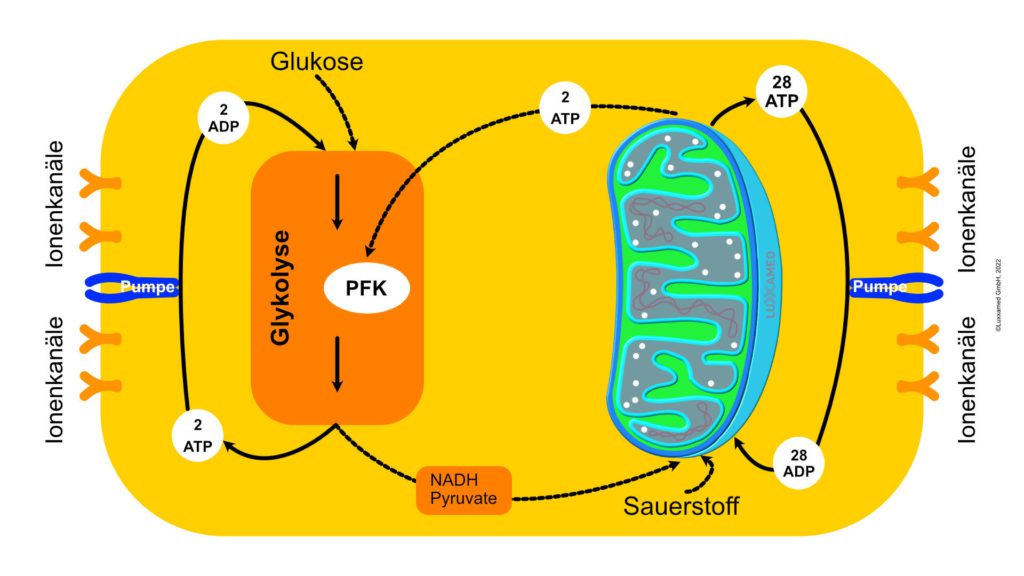
ATP is necessary for nerve transmission. Nerve transmission involves the release of nerve messengers from the presynaptic terminal into the synaptic cleft, which, simply put, is a space between one nerve and another. The nerve substance spans the gap and attaches to the receptor of the other cell. The nerve transmitter substance must be constantly formed in the presynaptic terminal for later release; the energy for this formation is provided by ATP. In the presynaptic terminal there are many mitochondria that form and store the ATP for this process. The formation of ATP will be discussed later in relation to the stimulatory effects of the microcurrent.
At the postsynaptic terminal, the next nerve cell, concentration gradients in the nerve cell membrane cause the nerve to fire and the nerve signals to travel to the next presynaptic terminal through the active transport of sodium, potassium and calcium. These concentration gradients could not be achieved without active ATPase transport across nerve cell membranes. Active transport is brought about by the release of energy from ATP as it splits its phosphate bonds (see Figure 2 for the chemical structure of ATP).

Active transport is a means of moving molecules against a concentration gradient across the cell membrane either into or out of the cell. This concentration gradient can be an electrical gradient or a pressure gradient. Sodium, potassium, calcium, glucose, amino acids and many other compounds are transported in this way.
In summary, ATP is the energy currency for our bodies. In fact, virtually every cytological, histological and physiological process is ATP-mediated, making ATP clinically important. Theoretically, our bodies can produce as much ATP as they need, but in reality they do not. Microcurrent therapy with a current of 200-800 microamperes is a way of charging the tissues with ATP, which remains there until it is needed. This explains much of the research showing an increase in the rate of healing.
In a clinical sense, any healing process requires a large amount of ATP and can be accelerated by increasing the ATP in the tissue. Microcurrent therapy achieves this by being able to increase ATP in tissues by up to 500%.
Microcurrent and ATP
.
Microcurrent application causes an increase in ATP production. This allows the body to accelerate the healing process it has initiated. It can even enable it to get over the proverbial “hump” that was out of reach due to insufficient ATP concentrations to make the necessary changes.
ATP is the dynamic energy reservoir in our body. Glucose serves as a longer-term reservoir, but in itself contributes relatively little to the body’s energy supply. Glucose is first converted into ATP. ATP is the storage and distribution vehicle for energy. From the moment an ATP molecule is produced, it is normally consumed within a minute.
The turnover rate of ATP is very high. However, the body has a large capacity to store ATP. You can build up ATP reserves. This is one reason why microcurrent, unlike other forms of electrotherapy such as interference current, TENS and galvanic with higher amperage, has a cumulative effect rather than a decreasing effect.
Furthermore, these devices cannot even be used in the area of ATP generation. Any stimulation greater than 1,000 microamps has been shown to plateau and then decrease ATP. Microcurrent therapy, used clinically in the 1 to 600 mA range, is the treatment of choice for improved tissue healing. Research and clinical trials have shown that microcurrent therapy reduces the healing time of ulcers and sprains/strains by 40-50%, fractures heal faster and stronger, and even bad scars (keloid scars) remodel into a healthier, stronger scar. Other ATP-related stimulating effects of microcurrent include reducing inflammation, oedema and swelling, and increasing physical endurance during exercise.
Clinically, microcurrent therapy is by no means limited to its effect of increased ATP production in its capacity as a treatment modality, but its effects on halving injury healing time are truly dramatic. The mechanism of increased ATP production by microcurrent stimulation can be explained. In the following text, the mechanism is shown from the crude external application down to the molecular level.
A microcurrent device delivers a pulsed direct current (DC) with variable square pulses so that the nature of the electrical flow is the same. Figure 3 shows a typical circuit in which electrons flow from the cathode to the anode, while current in the form of negative ions flows from the anode to the cathode. The negative ions can be thought of as the opposite pole to the electrons, which flow in the other direction. This completes the circuit.
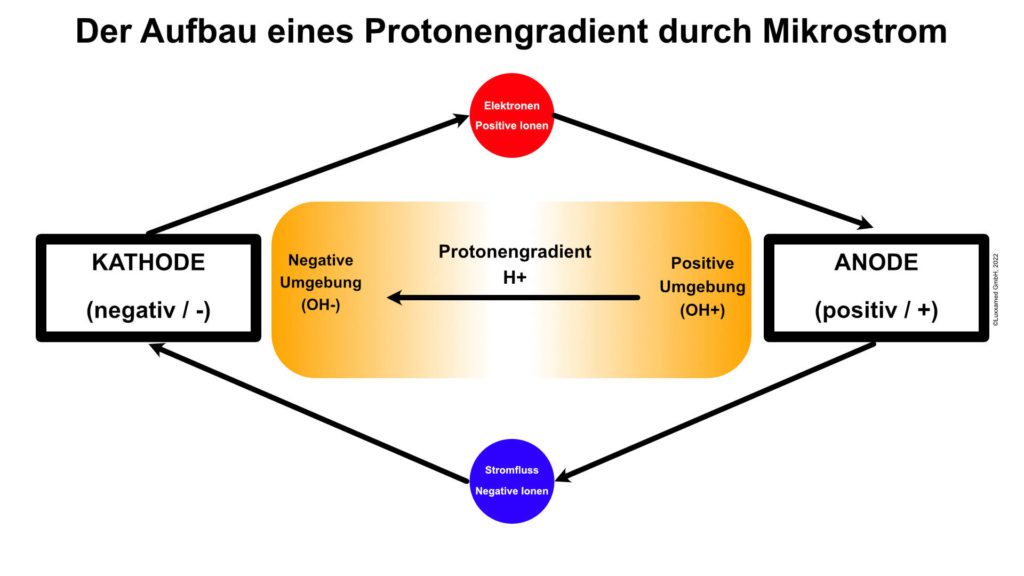
Of greater clinical relevance is what happens at the anode and cathode than the circuit itself. Around the cathode, which is negative, is an environment of negative hydroxyl ions (OH-). This is caused by the interaction of electrons with water molecules at the cathode, splitting the water molecules into hydrogen and hydroxyl molecules. The same reaction takes place at the anode; however, since the anode is positively poled, protons (hydrogen ions) form the environment of the anode.
At the moment, both hydrogen and hydroxyl ions form around the two pad electrodes. However, over time, the ionic environment around the anode electrode becomes hydrogen and around the cathode electrode becomes hydroxyl. Since it is the hydrogen that leads to the formation of ATP, it follows that, as a residual effect, ATP production continues at this point after the microcurrent stimulator is switched off.
At the negative electrode, on the other hand, ATP production stops immediately after the stimulator is switched off, as there is no residual hydrogen cloud in this area. Protons (H+) have a very strong effect here. In Figure 3 we see that the protons diffuse into an area with fewer protons, namely from the anode side to the cathode side. As the protons (H+) travel through the tissue, they cause increased formation of ATP.
This ATP formation can be explained by Mitchell’s chemosmotic theory. This theory explains how mitochondria form ATP through known processes such as the electron transport chain and the Krebs cycle. According to Mitchell’s theory, ionised hydrogen (protons) triggers the electron transport chain by combining with NADH to form NADH+ and with FAD to form FADH2 and other mediators. The net effect of each cycle of the electron transport chain is the introduction of six hydrogen ions between the inner and outer mitochondrial membranes.
At this point, the hydrogen ATPase is activated by the high levels of hydrogen in the membranes and ATP production is initiated. This occurs through the addition of a phosphate group to ADP to form ATP (see Figure 2, noting that the process is basically reversed to form ATP). This process is called oxidative phosphorylation. At this point, the ATP is transferred from the mitochondria to the cytoplasm of the cell, where it is stored until needed.
Cheng et. al (1982) – the ATP study on microcurrent therapy
.
Probably the most frequently cited study to explain or demonstrate ATP increase is Cheng et. al (1982). We have taken a closer look at this study and have prepared a summary for you.
Conclusion and afterthoughts
ATP can be produced by the body in many ways other than those mentioned above. However, it is a very dynamic source of energy and at the site of injury or overuse and micro-injury, ATP supply can be compromised.
Microcurrent therapy offers a unique and wonderful solution for tissue therapy. Clinically, microcurrent therapy is also a therapy of choice for acute injuries. It should also be remembered that in addition to its uniqueness, microcurrent therapy shares many of the characteristics of other electrical therapies: namely, its use for pain control, muscle relaxation and nerve regeneration. It is also unique in its ability to increase the permeability of blood vessels and its use as a means of electro-acupuncture. The microcurrent devices are capable of sensing the bioelectric state of the human body.
Sources
Dynamic Chiropractic 17 (18). Available online at https://www.dynamicchiropractic.com/mpacms/dc/article.php?id=36227, last checked 23/10/2022.
CHENG, NGOK; van HOOF, HARRY; BOCKX, EMMANUEL; HOOGMARTENS, MICHEL J.; MULIER, JOSEPH C.; DIJCKER, FRANS J. de et al. (1982): The Effects of Electric Currents on ATP Generation, Protein Synthesis, and Membrane Transport in Rat Skin. In: Clinical Orthopaedics and Related Research &NA; (171), 264-272. DOI: 10.1097/00003086-198211000-00045.
Mannheimer, Jeffrey S. (2005): The Effect of Microcurrent Stimulation on ATP Synthesis in the Human Masseter as Evidenced by 31P Magnetic Resonance Spectroscopy. Dissertation. Health Sciences Seton Hall University.
Wikipedia (2018): Adenosine triphosphate. Ed. v. Wikipedia. Available online at https://de.wikipedia.org/wiki/Adenosintriphosphat, last updated 28 Jan. 2018.
« Back to Glossary Index